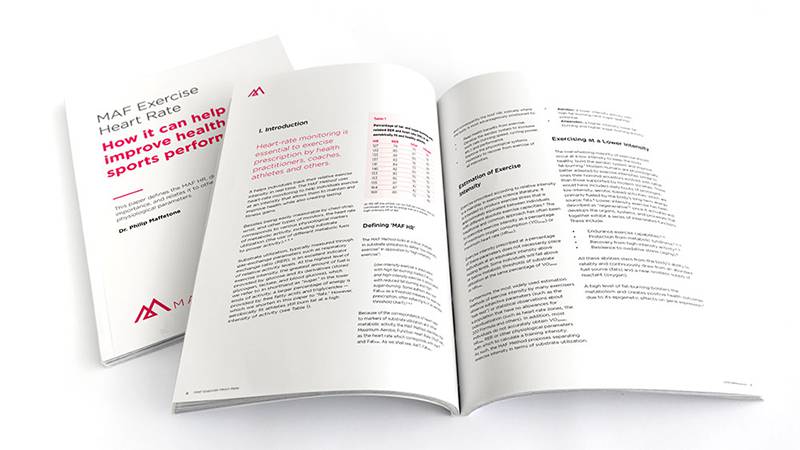
This paper defines the MAF HR, discusses its importance, and relates it to other standard physiological parameters.
Dr. Philip Maffetone
I. Introduction
Heart-rate monitoring is essential to exercise prescription by health practitioners, coaches, athletes and others. It helps individuals track their relative exercise intensity in real time. The MAF Method1 uses heart-rate monitoring to help individuals exercise at an intensity that allows them to maintain and improve health while also creating lasting fitness gains.
Besides being easily measurable by chest-strap, wrist, and other types of monitors, the heart rate corresponds to various physiological markers of metabolic activity, including substrate utilization (the use of different metabolic fuels to power activity).2,3,4
Substrate utilization, typically measured through gas-exchange parameters such as respiratory exchange ratio (RER), is an excellent indicator of relative activity levels. At the highest level of exercise intensity, the greatest amount of fuel is provided by glucose and its derivatives (stored glycogen, lactate, and blood glucose), which we refer to in shorthand as “sugar.” In the lower ends of activity, a larger percentage of energy is provided by free fatty acids and triglycerides — which we shorten in this paper to “fats.” However, aerobically fit athletes still burn fat at a high intensity of activity (see Table 1).
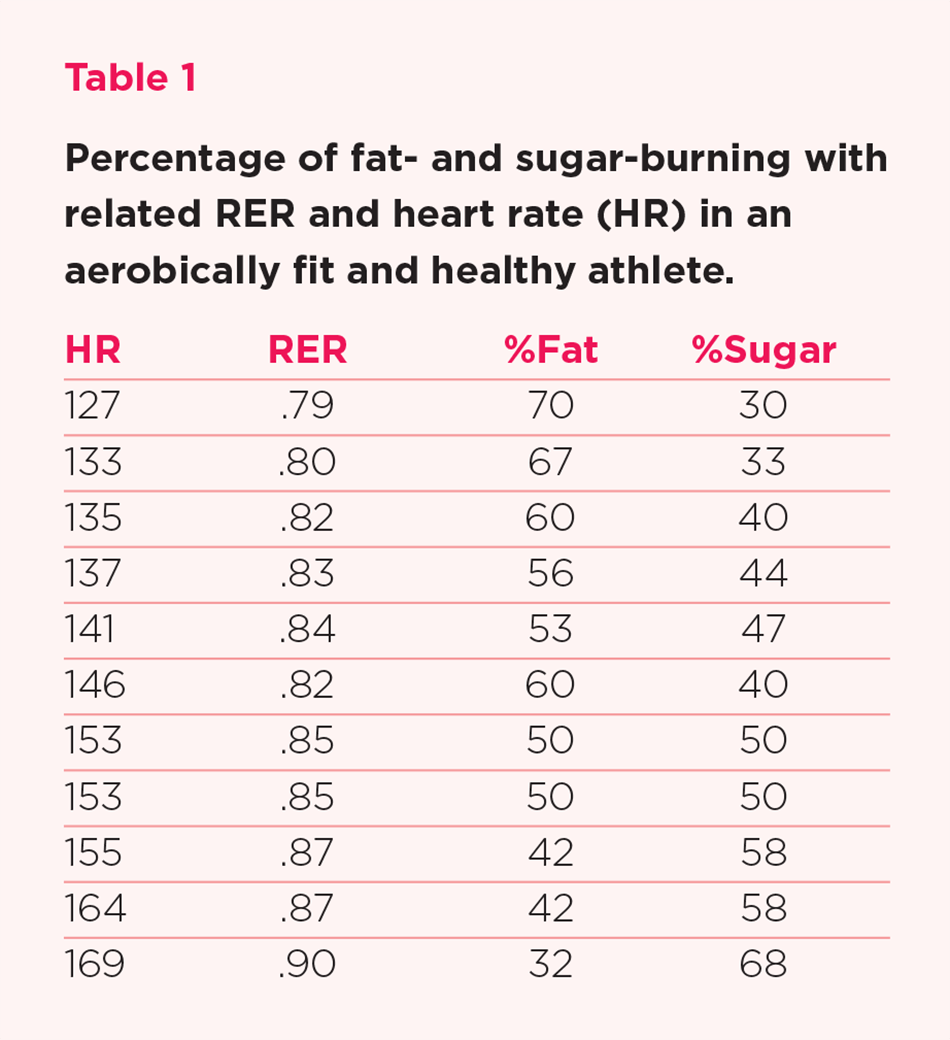
At 155 HR this athlete can run 5:25 per mile pace. Note the continued use of fat for energy even at a relatively high-intensity HR of 169.
Defining ‘MAF HR’
The MAF Method looks at critical changes in substrate utilization to define “low-intensity exercise” in opposition to “high-intensity exercise”:
Low-intensity exercise is associated with high fat-burning (called Fatmax), and high-intensity exercise is associated with reduced fat-burning and high sugar-burning. Some authors also use Fatmax as a threshold measure for exercise prescription, often referring to it as aerobic threshold (AerT).3,4,5
Because of the correspondence of heart rate to markers of substrate utilization and other metabolic activity, the MAF Method identifies the Maximum Aerobic Function Heart Rate (MAF HR) as the heart rate which corresponds with AerT and Fatmax. As we shall see, AerT, Fatmax, and consequently the MAF HR, indicate where the body is most advantageously positioned to:
- Reap health benefits from exercise.
- Develop the aerobic system to increase work rate (running speed, cycling power, etc.) and performance.
- Improve the physiological systems necessary to recover from exercise of
all intensities.
Estimation of Exercise Intensity
Exercise prescribed according to relative intensity is a mainstay in exercise science literature. It is intended to produce exercise stress that is approximately equivalent between individuals with different absolute exercise capacities.6 The traditional and common approach has often been to prescribe exercise intensity as a percentage of maximum oxygen consumption (VO2max) or maximum heart rate (HRmax).
Exercise intensity prescribed at a percentage of these parameters does not necessarily place individuals at an equivalent intensity above resting levels. Some individuals will fall above or below metabolic thresholds of substrate utilization at the same percentage of VO2max or HRmax.
Furthermore, the most widely used estimation methods of exercise intensity by many exercisers observe subjective parameters (such as the “talk-test”) or statistical observations about a population that have no allowances for individualization (such as heart-rate zones, the 220 Formula and others). In addition, most individuals do not accurately obtain VO2max, HRmax, RER or other physiological parameters with which to calculate a training intensity. As such, the MAF Method proposes separating exercise intensity in terms of substrate utilization:
- Aerobic: a lower-intensity activity with high fat-burning (and sugar-sparing) potential.
- Anaerobic: a higher-intensity, lower fat-burning and higher sugar-burning activity.
Exercising at a Lower Intensity
The overwhelming majority of exercise should occur at a low intensity to keep the body healthy, build the aerobic system and improve fat-burning.7 Modern humans are physiologically better adapted to exercise intensities similar to ones their hominid ancestors evolved with rather than those supported by modern societies. These would have included daily bouts of prolonged, low-intensity, aerobic-based activities, which are primarily fueled by the body’s long-term energy source: fats.8 Lower-intensity exercise has been described as “regenerative”3 since it activates and develops the organs, systems, and processes that together exhibit a series of interrelated functions.
These include:
- Endurance exercise capabilities.9,10
- Protection from metabolic syndrome.11,12,13
- Recovery from high-intensity activity.14,15
- Resilience to oxidative stress (aging).16
All these abilities stem from the body’s ability to reliably and continuously draw from an abundant fuel source (fats) and a near-limitless supply of reactant (oxygen).
A high level of fat-burning bolsters the metabolism and creates positive health outcomes due to its epigenetic effects on gene expression.17
The diverse mechanisms implicated in these abilities include the respiratory and cardiovascular systems (lungs, heart and blood vessels), but most importantly the slow-twitch aerobic (Type I) muscle fibers which, in addition to oxidizing fats,18 assist anaerobic (Type II) muscle fibers in their function during high-intensity efforts.14
High-intensity activity is associated with using a more powerful fuel (sugar) which is nevertheless much more limited than fats. Using sugar for energy allows the body to increase its energy production and work rate far beyond what the rate of oxygen uptake allows. When the rate of sugar usage exceeds the supply of oxygen, this sugar is burned anaerobically, or outside the presence of oxygen.
Anaerobic function creates higher levels of physical and biochemical stress,16 decreases immune function19, 20 and muscle repair,21 increases inflammation,22 increases the risk of muscle injury (most common in fast-twitch fibers),23 and impairs fat-burning.24 These conditions are also associated with poor (or a lack of) recovery, and are common components of and contributors to the overtraining syndrome.25
Greater fat oxidation is therefore a hallmark of low-intensity training and aerobic activity. It corresponds to a lower RER, and occurs at a lower percentage of maximum oxygen consumption (VO2max) than sugar-burning (typically around 75 percent, although health, fitness, age and other factors can raise or lower this number).3 As activity levels (and therefore RER and oxygen consumption) rise, so does sugar-burning.
Because anaerobic activity impairs fat-burning, we can extrapolate that Fatmax occurs just before the onset of anaerobic activity and the production of its main by-product, lactate.3 The onset of increasing lactate is also indicative of the start of glycogen depletion.26 As noted above, this point is referred to as the aerobic threshold (AerT) and Fatmax, which coincides with the MAF HR.
Calculating the MAF HR: The MAF 180 Formula
The AerT is located at an exercise intensity that is often described as a percentage of VO2max. This is sometimes referenced as 75 percent but it varies with and is largely determined by endurance training and subsequent fitness, health status and age.3,27 For example, AerT occurs at a lower percentage for those who are untrained, ill, or elderly. Values may be slightly higher for very well trained elite athletes (such as an AerT of 80%+ of VO2max), and lower for untrained individuals (AerT as low as 55% of VO2max). The AerT occurs at a higher percentage for adolescents, and at a lower percentage for those over age 60.28
After a few years of determining the MAF HR in individuals via an array of clinical assessments, including age, a comprehensive physical evaluation, gait analysis, health and fitness history, with confirmation using measures of gas exchange,29 it became clear that a heart rate equivalent to 180-age could constitute the beginnings of a potential formula for determining a person’s MAF HR (see Table 2). The 180 Formula indicates that certain modifications must be made depending on a person’s health and fitness status. These idiosyncrasies influence where the AerT and Fatmax, and therefore the MAF HR, will occur.
Table 2
The 180 Formula for determining MAF HR
Subtract your age from 180, then modify from one of the categories below:
- If you have or are recovering from a major illness (heart disease, any operation or hospital stay, etc.) or are on any regular medication, subtract an additional 10.
- If you are injured, have regressed in training or competition, get more than two colds or bouts of flu or other infection per year, have seasonal allergies or asthma, or if you have been inconsistent or are just getting back into training, subtract an additional 5.
- If you have been training consistently (at least four times weekly) for up to two years without any of the problems just mentioned, keep the number (180-age) as maximum.
- If you have been training for more than two years without any of the problems listed above, and have made progress in athletic competition without injury, add 5.
Exemptions:
The 180 Formula may need to be further individualized for athletes over the age of 65. For some, up to 10 beats may have to be added for those in category (d) in the 180 Formula, and depending on individual levels of fitness and health. This does not mean 10 should automatically be added, but that an honest self-assessment is important.
For athletes 16 years of age and under, the formula is not applicable; rather, a heart rate of 165 may be best.
Prescribing Aerobic Exercise
Speed or power at the MAF HR is an important physiological predictor of endurance performance. Studies show that submax thresholds are the best predictors of endurance performance in runners, cyclists, race walkers and other athletes, as well as in the performance of untrained people.30,31
The MAF Test was developed in order to track the improvement of the aerobic system across time (see Table 3). For a runner, this test may consist of a 3- to 5-mile run on an oval, 400-meter track, while recording the time per mile (or kilometer). The MAF Test should be preceded by a 15-minute warm-up and performed under consistent conditions (same shoes, weather, time of day, etc.) Other activities can also be used for the MAF Test, including cycling and rowing by measuring power, swimming by measuring laps, etc.
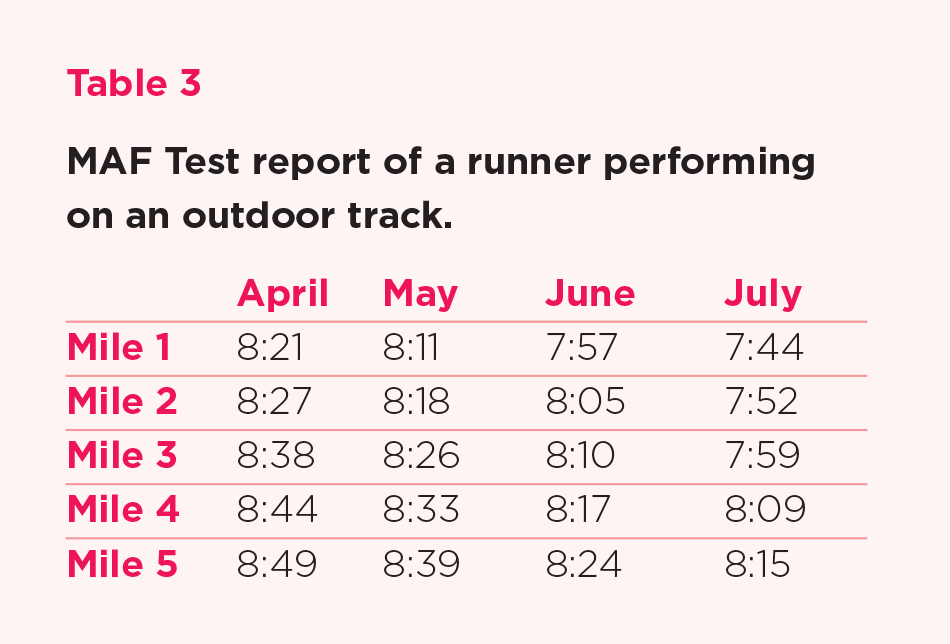
Increasing speed at the same submax HR translates to improvement in aerobic function and fat-burning, and can predict faster race performances.32 It has also been long known that aerobic contribution to energy during maximal exercise such as competition is significant, and increases with the duration of the event.30 See Table 4.
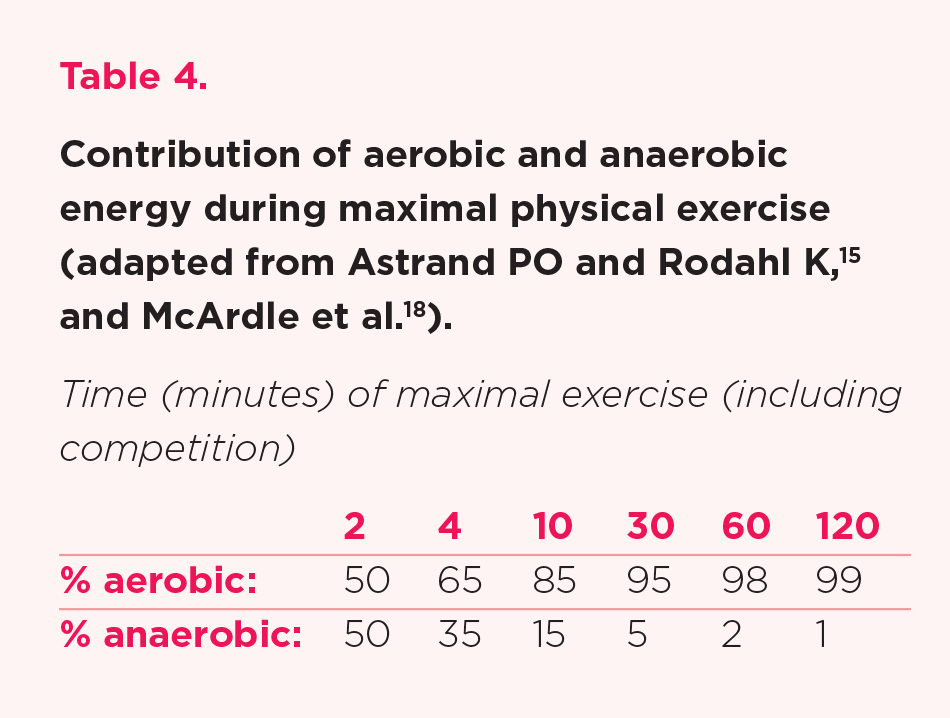
The 180 Formula is not a replacement for properly executed laboratory tests that determine the AerT, Fatmax and other metrics, although it usually corresponds with them. Given that the 180 Formula is applicable to a majority of the population, it can help individuals monitor workouts, improve fitness and build health. This makes it very useful to those who do not have access to regular laboratory testing.
References
- Maffetone P. White Paper. An Introduction to MAF: Maximum Aerobic Function. Independent; 2016.
- Montgomery P, Green D, Etxebarria N, et al. Validation of Heart Rate Monitor-Based Predictions of Oxygen Uptake and Energy Expenditure. J Strength Cond Res. 2009;23(5):1489-1495.
- Meyer T, Lucía A, Earnest C, Kindermann W. A Conceptual Framework for Performance Diagnosis and Training Prescription from Submaximal Gas Exchange Parameters: Theory and Application. Int J Sports Med. 2005;26:S38-S48.
- Kindermann W, Simon G, Keul J. The significance of the aerobic-anaerobic transition for the determination of work load intensities during endurance training. Eur J Appl Physiol. 1979;42(1):25-34.
- Londeree BR. Effect of training on lactate/ventilatory thresholds: a meta-analysis. Med Sci Sports Exerc. 1997;29(6):837-843.
- Mann T, Lambert RP, Lambert MI. Methods of prescribing relative exercise intensity: physiological and practical considerations. Sports Med. 2013;43(7):613-25.
- Maffetone P. Complementary Sports Medicine. Champaign, IL: Hum Kinet; 1999.
- Boullosa D, Abreu L, Varela-Sanz A, Mujika I. Do Olympic Athletes Train as in the Paleolithic Era? Sports Med. 2013;43(10):909-917.
- Hickson RC, Rennie MJ, Conlee R, et al. Effects of increased plasma fatty acids on glycogen utilization and endurance. J Appl Physiol. 1977; 43: 829-833.
- Holloszy JO, Coyle EF. Adaptations of skeletal muscle to endurance exercise and their metabolic consequences. J Appl Physiol. 1984; 56: 831-838.
- Ellis AC, Hyatt TC, Hunter GR, Gower BA. Respiratory quotient predicts fat mass gain in premenopausal women. Obesity. 2010; 18(12): 2255-2259.
- Mattson M, Allison D, Fontana L, et al. Meal frequency and timing in health and disease. Proc Natl Acad Sci. 2014; 111(47): 16647-16653.
- Schutz Y. Abnormalities of fuel utilization as predisposing to the development of obesity in humans. Obesity Res. 1995; 3(2): 173s-178s.
- Haseler LJ, Hogan MC, Richardson RS. Skeletal muscle phosphocreatine recovery in exercise-trained humans is dependent on O2 availability. J Appl Physiol. 1999; 86:2013-8.
- Astrand PO and Rodahl K. Textbook of Work Physiology. New York, McGraw-Hill 1977.
- Powers SK, Jackson MJ. Exercise-Induced Oxidative Stress: Cellular Mechanisms and Impact on Muscle Force Production. 2008. Physiol Rev. 2008;88(4): 1243-1276.
- Volek J, Noakes T, Phinney SD. Rethinking fat as a fuel for endurance exercise, Eur J Sport Sci. 2015; 15:1, 13-20.
- McArdle W, Katch F, Katch V. Exercise Physiology. 3rd ed. Philadelphia, PA: Lea & Febiger; 1991.
- Putman CT, Jones NL, Hultman E, et al. Effects of short-term submaximal training in humans on muscle metabolism in exercise. Am J Physiol. 1998;275:E132–E139.
- Walsh N, Gleeson M, Shepard R et al. Position statement part one: immune function and exercise. Immunol Rev. 2011;17:6-63.
- Szivak T, Hooper D, Dunn-Lewis C et al. Adrenal Cortical Responses to High-Intensity, Short Rest, Resistance Exercise in Men and Women. J Strength Cond Res. 2013;27(3):748-760.
- van de Vyver M, Engelbrecht L, Smith C, Myburgh K. Neutrophil and monocyte responses to downhill running: Intracellular contents of MPO, IL-6, IL-10, pstat3, and SOCS3. Scand J Med Sci Sports. 2015.
- Blankenbaker DG, De Smet AA. MR imaging of muscle injuries. Appl Radiol. 2004:14–6.
- Boyd A, Giamber S, Mager M, Lebovitz H. Lactate inhibition of lipolysis in exercising man. Metabolism. 1974;23(6):531-542.
- Kreher JB and Schwartz JB. Overtraining Syndrome. A Practical Guide. Sports Health. 2012 Mar; 4(2): 128–138.
- Billat V, Sirvent P, Py G, Koralsztein J, Mercier J. The Concept of Maximal Lactate Steady State. Sports Med. 2003;33(6):407-426.
- Emerenziani GP, Gallotta MC, Meucci M et al. Effects of Aerobic Exercise Based upon Heart Rate at Aerobic Threshold in Obese Elderly Subjects with Type 2 Diabetes. Int J Endocrinol. 2015 May 18;2015.
- Bassett DR, Howley ET. Limiting factors for maximum oxygen uptake and determinants of endurance performance. Med Sci Sports Exerc. 2000 Jan 1;32(1):70-84.
- Høeg T, Maffetone P. The Development and Initial Assessment of a Novel Heart Rate Training Formula. Poster presented at the Medicine & Science in Ultra-Endurance Sports 2nd Annual Conference; May 2015; Olympic Valley, CA, USA.
- Achten J, Gleeson M, Jeukendrup AE. Determination of the exercise intensity that elicits maximal fat oxidation. Med Sci Sports Exerc. 2002; 34: 92-97.
- Dantas JL, Doria C. Detection of the Lactate Threshold in Runners: What is the Ideal Speed to Start an Incremental Test? J Hum Kinet. 2015 Mar 1;45(1):217-24.
- Yoshida T, Chida M, Ichioka M, Suda Y. Blood lactate parameters related to aerobic capacity and endurance performance. Eur J Appl Physiol Occup Physiol. 1987;56:7–11.
Special thanks to Ivan Rivera for assistance in writing and editing, Hal Walter for editorial and Simon Greenland for formatting.