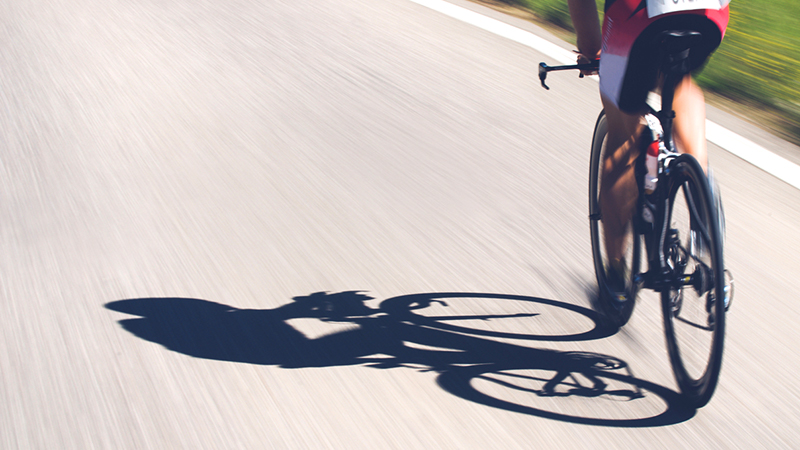
This original research paper has been accepted for publication in the July 2017 edition of International Journal of Sports Science and Coaching.
By Philip B. Maffetone-1 and Paul B. Laursen2,3
Abstract
While most endurance athletes and coaches recommend higher training loads and high-carbohydrate low-fat diets, some studies report benefits of lower intensity training for performance improvements and a low-carbohydrate high-fat eating plan. We present the case of a 38-year-old female professional/elite Ironman triathlete who presented with decreased performances, alongside severe gastrointestinal distress, excess fatigue, and severe daytime hunger. Training load was reduced from 30 to 18 h before a gradual increase to 24 h. Dietary recommendations included reducing carbohydrate and increasing dietary fat intake. Over a six-week period, daily carbohydrate content was gradually decreased from 73% (475 g) to 12% (78 g) of total calories, while fat content increased from 14% (40 g) to 75% (217 g), and protein levels remained constant at 13% (85 g). Within two months, the athlete reported increased perception of daily energy during and between training sessions, less perceived hunger and fatigue, and reduced need for daytime naps. Cycling power output increased by 20Wand run pace increased (12–15 s km-1) at the same training heart rate (141 beat min-1), and with the exception of water, nutrition was no longer required during long duration training (4 h). Race calories consumed were reduced from 400 kcal h-1 in previous years to 175, 145, and 130 kcal h-1 over the course of the next three Ironman events. Signs and symptoms of gastrointestinal stress ceased to occur, and two of three races were personal best times, with the final event completed in 8 h 52 min. We conclude that reducing training load, and dietary carbohydrates and increasing dietary fat, markedly improved a variety of health functions, reduced exogenous nutrient requirements during training and racing, and increased performance in an elite triathlete.
Key words
Ultraendurance performance, fatigue, gastrointestinal distress, nutrition, training load.
Introduction
This case study combines the art and science perspectives of a clinician/coach (PM) and an applied sport scientist/physiologist, with a combined experience in endurance sports of over 60 years. Our collaboration arises from a shared primary observation of the need to help recreational and professional/elite athletes maintain both health and fitness.¹ We define¹ these two terms separately as follows:
- Health: a state of complete, mental, social, and physical well-being, where all bodily systems (nervous, hormonal, immune, digestive, etc.) function in harmony.
- Fitness: the quality of human performance, especially in exercise and sports.
In a society promoting health, well-being, and physical performance, the paradoxical high rates of injury, illness, and even disease states in athletic populations2-4 seem unacceptable. In short, too many athletes are fit but unhealthy, with health-related conditions that adversely affect performance. In many cases, it can be the training load and food quality that influence both health and fitness.1
While high-intensity training is often considered the main stimulus for improvements in competitive endurance performance,5 some studies demonstrate that lower intensity training results in significant fitness improvements.6,7 For example, Esteve-Lanao et al.7 showed that endurance runners who trained in a polarized fashion,8 where 80% of their training time was conducted at a lower intensity and 20% of training time at a higher intensity, had significant 10km performance improvements over those training 35% of the time at higher intensities.
Few clinicians, sport scientists, and coaches recommend low-carbohydrate high-fat (LCHF) eating plans to improve athletic performance. Consensus statements from leading governing bodies generally recommend that carbohydrate quantities should range from 3 to 12 g kg1 body weight.9-10 However, recent work suggests that LCHF diets that raise blood ketone levels can increase fat oxidation rates and markers of health and exercise performance.11-12 In addition to increased fat oxidation, other potential benefits of LCHF eating plans for endurance athletes include improved training and racing energy, lowered incidence of delayed onset of muscle soreness, reductions in exogenous caloric requirements during training and competition, and the reduced incidence of serious gastrointestinal complaints.13 Although nontraditional, an LCHF eating plan approach has been recommended for athletes in a variety of sports for nearly 40 years,14 and this report is representative of other cases.
Case Report
A 38-year-old female professional/elite triathlete (and medical doctor) of 13 years presented with decreased performances in the Ironman triathlon, alongside a number of physiological ailments during training and competition over the previous several years. These included severe gastrointestinal distress (vomiting and bloody diarrhea several times during all previous Ironman events), excess fatigue and exhaustion, and severe daytime hunger not completely remedied with adequate energy intake. Menstrual cycles ranged from 33 to 37 days, with significant premenstrual syndrome and dysmenorrhea. A cholecystectomy was performed in 2013 without complications due to localized pain when eating fatty food. Upon initial presentation, fasting blood glucose was slightly elevated (103 mgdl-1) and all other laboratory test results were within normal limits. Following a clinical evaluation that included an extensive medical and training/racing history, and neuromuscular examination, training and treatment goals were directed at addressing the abovementioned problems.
The patient’s height was 165 cm and at the start of 2015 monthly body weight ranged from 60 to 61 kg. Following a review of the athlete’s dietary habits and food frequency, including a four-day detailed food record, dietary recommendations were made and included eating ad libitum and eliminating refined carbohydrates in daily meals. Guidelines also included helping the athlete determine the best balance of natural dietary carbohydrate and fat (while maintaining current protein intake) in alignment with naturally fluctuating energy/fatigue and hunger. In late January 2015, these dietary changes were initiated with the athlete assessing training energy, the need for exogenous training nutrition during long workouts, fatigue, hunger, and gastrointestinal comfort. Breath ketone (acetone) levels (Ketonix AB, Varberg, Sweden) were measured during daytime hours at rest. Data were recorded by the athlete, who provided daily and or weekly reports.
Training metrics were assessed daily with the use of a heart rate (HR) monitor and power meter, including average cycling power (watts) relative to mean submaximal HR during long rides that initially lasted 4h, running pace relative to the same mean submaximal HR during workouts lasting from 45 min to 2 h.
Training was completed as per recent years, except that both total workout volume and training intensity were reduced. Total weekly training duration was initially lowered from 30 to 18 h (approximately 4 h swimming, 8 h cycling, 6 h running), before a gradual increase in training volume to about 24 h of total training per week over the subsequent three months. Overall training intensity was reduced slightly to a mean HR of 141 beat min1, while high-intensity training was discontinued.
It is important to note that this case study is of a patient (of PM’s), and a clinician’s job is to help the patient improve her health and fitness, and only secondarily would use the information in a scientific format such as this retrospective case report. It would, in fact, be an ethical conflict for a clinician to withhold therapeutic recommendations over the course of a doctor– patient relationship for reasons of not comparing the effect of training intensity versus dietary influencers on health. The same potential conflict is also the reason why various other tests were not performed—they were not deemed clinically necessary for proper care of the patient at the time, despite the fact that these could have improved the study design and learning outcomes.
Results
The daily macronutrient makeup of the athlete’s 2700 kcal diet (monitored by the subject, experienced with dietary assessment) gradually changed over an initial six-week period, with carbohydrate content decreasing from 73 to 12%, fat content increasing from 14 to 75%, and protein levels remaining constant at 13% (Figure 1). There was a slight (100 kcal) reduction in the total calories over this period. Nutrient amounts included those consumed during long training sessions (noted below). These dietary changes were reported to be well tolerated.
Within two month’s modification of the training and eating changes, the athlete reported increased perception of daily energy during and between training sessions, less perceived hunger and fatigue, and reduced need for daytime naps. Breath ketone measurements, not available prior to the start of 2015, began rising after approximately three weeks, remaining in the mild to moderate ketosis range throughout most of the season. Cycling power increased by 20Wand run pace increased (12–15 s km-1) at the same HR (141 beat min1) during training sessions by October 2015.
The need to consume food and drink (except water) during long duration training gradually decreased after about eight weeks. Long rides up to 4 h at the training HR (141 bpm) power output no longer required exogenous nutrient supply to sustain energy and power. During long weekly cycling sessions (up to 7 h) food intake usually began around hour 4, averaging 100–200 kcal h-1 and included a fat-to-carbohydrate ratio that ranged from 50:50 to 10:90. Foods included applesauce, dried fruit, energy bars, and honey-based fudge, along with a mixture of honey and coconut oil. Most carbohydrates were in monosaccharide form.
Figure 1.
Daily macronutrient intake before and after dietary shift and intervention.
Within three months of dietary changes, body weight was reduced to 55 kg, and later in the year rose marginally (56–57 kg). The patient reported being more lean, with clothing fitting noticeably looser (in particular, waist size). By the end of 2015, the patient reported menstrual cycles at 31–33 days, with a significant reduction in dysmenorrhea and an almost asymptomatic premenstrual state.
No significant physical injuries (complaints of physical pain or disability lasting more than a few days) were reported during 2015. By January 2016, fasting blood glucose was normal (91 mg dl-1).
Race preparation and results
A prerace taper with reductions in training volume of 60–70% was implemented beginning two weeks before the day of each race, before a slow rebuilding of training volume over the 2–3-week period following the first two events.
Three international Ironman competitions were successfully completed during 2015, in May, August, and November (Table 1). As determined during longer race-specific training sessions, prerace meals for these events contained approximately 20 g carbohydrates, 20 g protein, and 100 g fat (total 1060 kcal). Race calories consumed, based on the athlete’s subjective requirement for exogenous nutrients, were reduced from 400 kcal h1 in previous years to 175, 145, and 130 kcal h1 over the course of the next three Ironman events (Figure 2). Signs and symptoms of gastrointestinal stress, as reported in previous events, ceased to occur. Two races (Brazil Ironman, Arizona Ironman) were personal best times with the final event completed in 8 h 52 min (Table 1). The athlete’s first 2015 Ironman race (Brazil) under the new diet and training strategy was a personal best time by 7 min.
Figure 2.
Caloric intake rates during Ironman triathlon event competitions.
Discussion
This case report describes the health and performance challenges experienced by a 38-year-old female professional/elite Ironman triathlete, presenting with reduced exercise performances concomitant with gastrointestinal distress, fatigue, hunger, and premenstrual syndrome with dysmenorrhea. The progressive adjustment of her training load, which included reductions in volume and intensity, and her nutrition plan, specifically the macronutrient ratio balance toward nutritional ketosis (LCHF), was associated with an alleviation of her presenting symptoms, reduced daily and exercise caloric requirements, and personal best Ironman performances.
Many of this athlete’s signs and symptoms may be associated with the overtraining syndrome.15–17 The reduction in training load and or increased energy availability is consistent with interventions that have been shown to be effective in treating athletes suffering from overtraining.
High-intensity training is traditionally considered the primary approach for improvements in competitive performance.5 However, some studies demonstrate that lower intensity training also results in fitness improvements in endurance athletes.6,7 Endurance competitions such as the Ironman triathlon do not rely on maximal efforts, but instead are typically much lower, such as 70–75% of VO2max. Therefore, training mostly the capacity to work at submaximal exercise intensities can improve the oxidation of fats and the performance of the slow-twitch aerobic muscle fibers, both of which may be of most importance for endurance success.18
There are also increasing numbers of reported performance benefits of lowering dietary carbohydrate.12,19,20 Phinney et al.21 showed enhanced fat oxidation rates in cyclists who reduced dietary carbohydrate to less than 50 g per day and substituted calories with dietary fat over four weeks. Enhanced lipid oxidation during exercise, drawing on a significant fuel reserve even in the leanest of individuals, spares limited muscle and liver glycogen, and can be oxidized at high rates in highly trained individuals.6 Moreover, training under conditions of low glycogen (termed ‘‘training low’’) enhances training adaptation, through greater AMPK signaling, with downstream effects on mitochondrial biogenesis, fat oxidation, and endurance performance.12,19,20 While acknowledging that training during periods of low carbohydrate availability and low glycogen levels can also enhance fat oxidation rates,22,23 these strategies might not induce other possible benefits of LCHF eating plans.13
Moreover, we have recently proposed the existence of an underreported number of ‘‘fit but unhealthy athletes’’ today.1 We hypothesize that this may be due to two primary drivers: an excessive volume of high training intensity coupled with a highly processed, high-glycemic diet. Both factors may elicit a sympathetic and hypothalamic–pituitary–adrenal (HPA) axis response, which drive increased systemic reactive oxygen species production, chronic inflammation, and a metabolic substrate shift toward carbohydrate and away from fat oxidation, manifesting in an array of signs and symptoms often labeled as the overtraining syndrome.16
A possible reason for the alleviation of the athlete’s symptoms in this case study (gastrointestinal distress, fatigue, hunger, and menstrual complaints) in response to the implementation of the exercise and dietary recommendations may relate to the removal of physiological stress on the HPA axis, stemming largely via the improved whole body fat oxidation rates. Changes in this athlete’s menstrual function may have been associated with improvements in the HPA (and or -ovarian) axis and increase in energy from fat oxidation. 24 Increased dietary fat intake may improve the sex hormone profile by raising testosterone and progesterone levels.25
Due to the initial presentation of premenstrual syndrome, dysmenorrhea, and elevated fasting blood glucose, a diagnosis of polycystic ovary syndrome was considered (for which treatment may include dietary carbohydrate reduction), and further evaluation was deemed unnecessary at the time.
While the measurement of respiratory exchange ratio (RER) was not available, fat oxidation appeared to increase based on the above-mentioned changes in signs and symptoms of health and performance. Despite the lack of RER data for this athlete, increased fat oxidation has been shown to correlate with better health outcomes, including reduction of excess weight and fat stores, increased energy and even lower disease risk, as well as increased endurance performance.20,26,27
The athlete’s pre-2015 dietary intake was only 100 kcal more than her post-2015 dietary intake, and combined with reduced training and the self-reported loss of 5 kg, the athlete may have previously been in a state of low energy availability. It is also possible that the patient’s likely increased fat oxidation rates may have contributed to her improved metabolism and may be aligned with her reported reduction in waist size.
Endurance athletes frequently report gastrointestinal impairment during competition, which can include dangerous and debilitating conditions such as nausea, bloating, pain, vomiting, abdominal angina, bloody diarrhea, endotoxemia, hemorrhagic gastritis, and ischemic colitis.28,29 Causes of gut distress are considered multifactorial, with gastrointestinal ischemia being important, along with mechanical position (e.g. runners versus cyclists) and nutrition. The complete alleviation of gastrointestinal ailments in this patient may have contributed to improved performance. The reduced exogenous caloric needs may have been due to higher fat oxidation rates and could have also contributed to improved performance.
Summary/conclusion
This case study outlines the long-term progression of an athlete’s adoption to reduced training load and an LCHF ketogenic eating plan. These shifts in behavior resulted in improvements in various health factors, including gastrointestinal and menstrual function, along with reduced caloric needs during racing, and increased performance.
Declaration of Conflicting Interests
The author(s) declared no potential conflicts of interest with respect to the research, authorship, and/or publication of this article.
Funding
The author(s) received no financial support for the research, authorship, and/or publication of this article.
References
- Maffetone P and Laursen PB. Athletes: fit but unhealthy? Sports Med Open 2015; 2: 24.
- Schnohr P, O’Keefe JH, Marott JL, et al. Dose of jogging and long-term mortality: the Copenhagen City Heart Study. J Am Coll Cardiol 2015; 65: 411–419.
- Buchheit M, Simpson BM, Schmidt WF, et al. Predicting sickness during a 2-week soccer camp at 3600 m (ISA3600). Br J Sports Med 2013; 47: i124–i127.
- Andersen CA, Clarsen B, Johansen TV, et al. High prevalence of overuse injury among iron-distance triathletes. Br J Sports Med 2013; 47: 857–861.
- Gibala MJ and McGee SL. Metabolic adaptations to short-term high-intensity interval training: a little pain for a lot of gain? Exerc Sport Sci Rev 2008; 36: 58–63.
- Robinson DM, Robinson SM, Hume PA, et al. Training intensity of elite male distance runners. Med Sci Sports Exerc 1991; 23: 1078–1082.
- Esteve-Lanao J, Foster C, Seiler S, et al. Impact of training intensity distribution on performance in endurance athletes. J Strength Condit Res 2007; 21: 943–949.
- Seiler S. What is best practice for training intensity and duration distribution in endurance athletes? Int J Sports Physiol Perform 2010; 5: 276–291.
- Thomas DT, Erdman KA and Burke LM. Position of the academy of nutrition and dietetics, dietitians of Canada, and the American College of sports medicine: nutrition and athletic performance. J Acad Nutr Diet 2016; 116: 501–528.
- Thomas DT, Erdman KA and Burke LM. American college of sports medicine joint position statement. Nutrition and athletic performance. Med Sci Sports Exerc 2016; 48: 543–568.
- Noakes T, Volek JS and Phinney SD. Low-carbohydrate diets for athletes: what evidence? Br J Sports Med 2014; 48: 1077–1078.
- Volek JS, Freidenreich DJ, Saenz C, et al. Metabolic characteristics of keto-adapted ultra-endurance runners. Metabolism 2016; 65: 100–110.
- Volek JS, Noakes T and Phinney SD. Rethinking fat as a fuel for endurance exercise. Eur J Sport Sci 2015; 15: 13–20.
- Maffetone P. Complementary sports medicine. Champaign, IL: Human Kinetics, 1999.
- Meeusen R, Duclos M, Foster C, et al. Prevention, diagnosis, and treatment of the overtraining syndrome: joint consensus statement of the European College of Sport Science and the American College of Sports Medicine. Med Sci Sports Exerc 2013; 45: 186–205.
- Kreher JB and Schwartz JB. Overtraining syndrome: a practical guide. Sports Health 2012; 4: 128–138.
- Schmitt L, Regnard J and Millet GP. Monitoring fatigue status with HRV measures in elite athletes: an avenue beyond RMSSD? Front Physiol 2015; 6: 343.
- Laursen PB. Training for intense exercise performance: high-intensity or high-volume training? Scand J Med Sci Sports 2010; 20: 1–10.
- Bartlett JD, Louhelainen J, Iqbal Z, et al. Reduced carbohydrate availability enhances exercise-induced p53 signaling in human skeletal muscle: implications for mitochondrial biogenesis. Am J Physiol Regul Integr Comp Physiol 2013; 304: R450–R458.
- Hetlelid KJ, Plews DJ, Herold E, et al. Rethinking the role of fat oxidation: substrate utilisation during high-intensity interval training in well-trained and recreationally trained runners. BMJ Open Sport and Exerc Med 2015; Doi: 10.1136/bmjsem-2015-000047.
- Phinney SD, Bistrian BR, Evans WJ, et al. The human metabolic response to chronic ketosis without caloric restriction: preservation of submaximal exercise capability with reduced carbohydrate oxidation. Metabolism 1983; 32: 769–776.
- Marquet LA, Brisswalter J, Louis J, et al. Enhanced endurance performance by periodization of carbohydrate intake: ‘‘sleep low’’ strategy. Med Sci Sports Exerc 2016; 48: 663–672.
- Yeo WK, Paton CD, Garnham AP, et al. Skeletal muscle adaptation and performance responses to once a day versus twice every second day endurance training regimens. J Appl Physiol 2008; 105: 1462–1470.
- Loucks AB and Redman LM. The effect of stress on menstrual function. Trends Endocrinol Metab 2004; 15: 466–471.
- Mumford SL, Chavarro JE, Zhang C, et al. Dietary fat intake and reproductive hormone concentrations and ovulation in regularly menstruating women. Am J Clin Nutr 2016; 103: 868–877.
- Ellis AC, Hyatt TC, Hunter GR, et al. Respiratory quotient predicts fat mass gain in premenopausal women. Obesity 2010; 18: 2255–2259.
- Mattson MP, Allison DB, Fontana L, et al. Meal frequency and timing in health and disease. Proc Natl Acad Sci USA 2014; 111: 16647–16653.
- Pfeiffer B, Stellingwerff T, Hodgson AB, et al. Nutritional intake and gastrointestinal problems during competitive endurance events. Med Sci Sports Exerc 2012; 44: 344–351.
- de Oliveira EP, Burini RC and Jeukendrup A. Gastrointestinal complaints during exercise: prevalence, etiology, and nutritional recommendations. Sports Med 2014; 44: S79–S85.
Reviewers:
Andrew Bosch (University of Cape Town, South Africa) Gre´goire Millet (Institute of Sport Sciences, Switzerland) Daniel Plews (High Performance Sport New Zealand, New Zealand)
- Athletic Consultant, Arizona, USA
- Sports Performance Research Institute New Zealand (SPRINZ), AUT University, Auckland, New Zealand
- High Performance Sport New Zealand, Auckland, New Zealand
Corresponding Author:
Paul B. Laursen
High Performance Sport New Zealand
PO Box 302 563, North Harbour, Auckland 0751
New Zealand
Tel: +64 21 303 153
E-mail: paul.laursen@hpsnz.org.nz